Non-laser Light Sources: Ultraviolet LEDs illuminate the scientific landscape
With differences in spectral distribution, power level, and regulatory issues, UV LEDs challenge mercury arc lamps for disinfection, chromatography, and fluorescence imaging applications.
Ultraviolet (UV) light-emitting diodes (LEDs) have advanced in recent years beyond their initial use in UV curing applications to reach illumination levels that allow them to challenge incumbent mercury-containing UV arc lamps. The life sciences market is now hungry for UV light sources for disinfection, chromatography, fluorescence imaging, and other emerging applications.
With differences in spectral distribution, power level, regulatory issues, and other parameters, the answer to the question of whether UV LEDs will ever fully replace UV mercury arc lamps is not easy to answer.
LEDs vs. lamps
UV LEDs are solid-state devices that produce light when electrical current flows from the positive (p-type or anode) side of a semiconductor circuit to the negative (n-type or cathode) side, creating a p-n junction. Each UV LED emits a narrow bandwidth of light at the junction where positive doped semiconductor holes join with negative electrons when voltage is applied.
Alternatively, traditional UV mercury arc lamps use an electric arc inside an ionized mercury gas to excite atoms that then decay, emitting photons. Microwave lamps excite the gas via microwave emission. And while xenon lamps use xenon gas (no mercury), they can only operate in “flash” mode rather than continuous-wave (CW) emission.
If engineered properly, UV LED semiconductor sources last beyond 20,000 hours of operating time, whereas traditional UV lamp lifetimes are around 9000 hours—less than half the lifetime of LED sources.
The sun is a source of the full spectrum of UV radiation, which is commonly subdivided into UV-A (315–400 nm), UV-B (280–315 nm), and UV-C (200–280 nm) light. Typically, UV LEDs have a narrow spectral output centered around a specific wavelength, ±10 nm.
Traditional UV applications
Many applications across the UV spectrum use either traditional lamps or UV LED light sources (see Fig. 1). The highest penetration and usage of UV LEDs is in adhesive curing applications—however, applications such as disinfection, chromatography, and fluorescence imaging continue to emerge as the technology evolves. With advantages in reliability, lifetime, instant on-off, and lower operating temperature, UV LED solutions are successfully replacing mercury lamps in numerous applications.
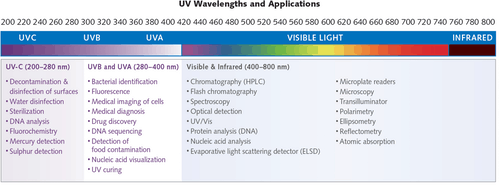
For industrial UV-curing applications, for example, most material companies have modified inks, coatings, and adhesives to support the narrow wavelengths for UV LEDs. However, surface curing has been a challenge. Fortunately, deep-UV (UV-C) LED irradiance and power continues to improve over time, increasing the ability of UV LEDs to replace traditional mercury lamps even in the most challenging surface cures.
Decontamination and disinfection
How effective are UV LEDs for inactivating (making inactive) biomolecules and microorganisms? The answer lies in the spectral intensity and dosage of the UV source. For example, UV-C light is known as “germicidal UV” for its effectiveness in decontamination and disinfection (see Fig. 2). While certain wavelengths affect different bonds within biological molecules, both nucleotides and proteins can be modified by deep-UV light. In short, both microorganisms and biological materials can be inactivated with the right dose of light.
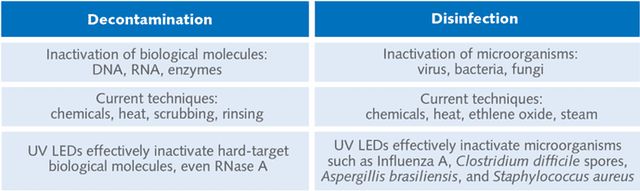
High-intensity UV LED technology offers unmatched levels of deep-UV irradiance compared to lamps, offering improved capabilities for decontamination and disinfection applications that require short wavelengths.
Enabling complete inactivation of contaminants in minutes, high-irradiance UV LED technology is currently being used by research labs and manufacturing facilities for inactivating biological molecules like DNA and RNA, as well as microorganisms.
Hard targets like RNase A—harmful to the upper respiratory system and mucous-membranes—can be completely inactivated with the right wavelength and intensity of UV light. By targeting specific molecular bonds, UV LED technology exhibits greater efficacy with lower total power consumption than broadband sources such as mercury lamps. Complete inactivation of laboratory contaminants can be accomplished by UV LEDs in less than five minutes and at a fraction of the cost of traditional methods.
Research from Phoseon (Hillsboro, OR) on the use of high-irradiance UV LED light engines for enzyme inactivation show that both irradiance (intensity) and radiant fluence (dose) contribute to rapid inactivation of the RNase A enzyme (see Fig. 3).

Ultraviolet light at 275 nm is thought to act on RNase A via an effect on the aromatic amino acids proximal to disulfide bonds. The 365 nm wavelength is targeted to the lysine side chain with the intent of destabilizing the RNase A reaction pocket. These two wavelengths interact synergistically to inactivate RNase A faster and more completely than either can alone. The research supports using high-intensity UV LED irradiation as a novel, fast, and convenient irreversible inactivation method for RNases on surfaces.
Chromatography and spectroscopy
Both deuterium lamps and UV LED light sources can be used as detection systems for chromatography and analytical instruments. Solid-state LED-based UV detectors deliver higher sensitivity and/or dynamic range, lower noise, and cooler, more-controllable operation than deuterium lamps and are significantly more stable. They turn on in milliseconds to full brightness, whereas conventional optical detection systems are bulky and slow to start, due to the arc lamps used as light sources. As a boon to users, solid-state light sources last 10,000 or more hours, compared to only 2000 hours for deuterium lamps.
Autofluorescence imaging
Barrett’s esophagus is a precancerous condition where epithelial cells of the lower esophagus change morphology to resemble the epithelial cells of the small intestine. Early neoplasia can be difficult to detect using conventional white-light endoscopy—in effect, screening of Barrett’s esophagus currently requires time-consuming biopsy and pathology.
In a move towards a real-time imaging system for Barrett’s morphology, Phoseon used 275 and 365 nm UV LEDs to excite tissue autofluorescence. The lining of porcine esophageal and duodenal tissues was used as a first-step model for the changes apparent with the characteristic Barrett’s transition to a more intestinal lining phenotype. Images showing easily visible differences in autofluorescent wavelength and intensity were captured using an Apple iPhone CMOS camera and analyzed.
A simple RGB image analysis of UV LED illuminated lumen tissue can provide a baseline for tissue discrimination between esophageal and duodenal tissue. While autofluorescent tissue discrimination is possible with 365 nm excitation alone, results improve dramatically when 275 nm illumination is added.
Tags: Chromatography & spectroscopy | Autofluorescence imaging | UV LED for Life Sciences | Non-laser Light Sources | Decontamination & disinfectionCategories: Decontamination & disinfection | Autofluorescence imaging | Chromatography & spectroscopy